Complex Systems Science and Ecological Resilience
Applying ecological principles to solution-building
Complex systems science as a formalized area of study is relatively new, or wildly old, depending on when you’d like to define its founding. Reductionist theory has a background in Greek philosophy-- particularly Descartes & Kant, with the general idea that everything is the sum of its parts.1 The intuitive response to this development would be that previously, complex systems were understood and were often explored using vague concepts such as the “natural way” or “God’s will”, and it was only by critiquing this understanding of cause and effect that the atomization of Reductionist theory could be created. While Reductionism offers a number of benefits, for us to understand the interconnected world around us, we rely specifically on complex systems theory to address complex, multifaceted issues. Simply put, sometimes things are more than the sum of their parts, such as we see in things like symbiotic relationships.
After centuries of reductionism driving scientific thought, folks in the early 20th century found themselves feeling limited by these types of inquiry, and what they realized was that we needed to move away from reductionist thinking in some forms and what came forward was the Macy conference in 1941, where specialists in a spectrum of scientific fields came together to talk science.2
It became apparent early on that they didn’t have the language to communicate across disciplines, despite the fact that on its surface, each specialist was working with the same ‘tools’ to understand the science they were studying. This is also exactly what made this conference so important. Similar patterns had emerged across disciplines, from ecologists to sociology, and it was there that the same patterns and processes were seen across the spectrum, and a new paradigmatic approach came out of it called ‘chaos theory’-- a direct challenge to linear systems, which were framed on the individualistic understanding of cause and effect extrapolated from Reductionist theory. 3
Later the name was changed to complex systems science, once they realized what they were witnessing wasn’t chaos, but rather larger models of something of a linear system-- in that, what was happening could be measured and predicted on larger scales, but on a smaller scale the amount of activity was overwhelming and immeasurable. 4
The great thing about complex systems science is that the same principles apply to any system-- it’s easy to move from one system to another and even across disciplines and be able to predict results.5 Linear systems, just like their name suggests, is the domino effect. Complex systems are the opposite-- parts don’t interact in any linear or consistent way, meaning that as these different parts act in different ways, they can change the system as a whole-- the system is dynamic-- it cannot be static. Think of a forest-- depending on the weather will impact the amount of acorns a tree produces, which impacts how many squirrels who live there, which impacts other animals, as well as the forests other animals previously lived in, and so on. Everything impacts other things and does not have a linear change impact. This makes it incredibly difficult to extrapolate specific data from specific inputs.
How much impact did increased rain have on a forest in one particular year? Well, if it is a mast year, its water needs are entirely different than typical years, and depending recent deer hunting tags, grazing pressure might change the chemical composition of the leaves of the species in order to become less palatable, which requires more energy, and suddenly you can see how quickly a question about rainfall ends up being predicated by a discussion of how covid has changed political support for deer hunting in a rural community.
This was the first big shift in fundamentally changing how research was done. Now, we see these systems nested within larger systems, all with the same general rules. The same way we can say a family has the same dynamics of a small town which is part of a metropolitan, and so on and so on.
Complex Systems Science & Climate Change
We can take this general framework and look at the biggest system of all that we are trying to tackle today; climate change. But to understand this, we need to have a comprehensive understanding of the subject before being able to engage with it through the lens of complex systems science. You may not realize this, but we are currently in an ice age-- we have been for about 10-15 million years.6 An ice age is defined when there is ice on one or both poles of the planet, which we technically have-- for now. During the last million years of this ice age a pattern has emerged every 100,000 to 120,000 years when we have had periods of vast glaciation, followed by quick periods of warming up, melting that ice away, and this pattern seems to be getting stronger and stronger and science isn’t quite sure why. 18,000 years ago we were at the last glacial maximum, called the Younger Dryas, which we are now receding from, a period which is called an interglacial period, and we should be going back into another glaciation period in the future.
Back to that glacial maximum 18,000 years ago, the Younger Dryas, a period during which the arctic ice cap reached down to long island and cape cod-- the period we generally think of when we speak about the ‘ice ages’. The average temperature was 51 degrees F. It’s important to remember this, because the temperatures we have experienced during modern human history, the last 2,000 years, is not the ‘typical’ temperature of the planet. 7 4% of the time over the last 2 million years are temperatures warm enough for the New England states, for example to have trees on them. 8
So, why does this happen? Why would the planet randomly warm up shortly, and then cool back down? It all has to do with our relationship to the sun. There are three pieces to this. One is a 100,000 year cycle where the orbit of the Earth going around the sun changes from circular to elliptical. 100,000 years is about the time it takes to go from circular, to elliptical, back to circular. The closer we are to the sun, obviously, the more warm we are. The second is regarding the axis tilt of the planet-- it adjusts from about 24.5 degrees to 22.5 degrees and back to 24.5 degrees every 40,000 years. The stronger the tilt, the more extreme the weather will be. The last piece is a 20,000 year cycle, where, do to the gravitational pressure of the planets in the solar system, the planet turns on a wobble, which impacts when the northern hemisphere is facing the sun-- whether it’s during the summer or the winter. The obvious impact of that is either warmer winters/cooler summers, or more extreme seasons. In 9,000 years, we will be closest to the sun during summer time, meaning right now we are experiencing more mild seasons, being at the middle of that cycle.9
Now, when we are in an elliptical pattern, meaning warm weather from the orbit, as well as a 24.5 degree axis tilt, meaning more extreme heat, and the wobble cycle positively impacting the northern hemisphere, meaning again-- more heat, we get these interglacial periods. There are three things that need to align to get enough sun gain to warm the planet.
Fundamentally, all of these parts have to align. We had all this align 6,000 years ago, and we reached the warmest temperature as a mean global temperature of 60 degrees. That’s a whopping 9 degrees warmer than the average temperature of the last ice age, let’s remember that. It took 12,000 years, with the perfect alignment of resources, to increase 9 degrees, and that was the most rapid climate change experienced on the planet in probably 65 million years, since the dinosaurs ruled the Earth and the planet was tropical. We have gone up a degree within 100 years. 10
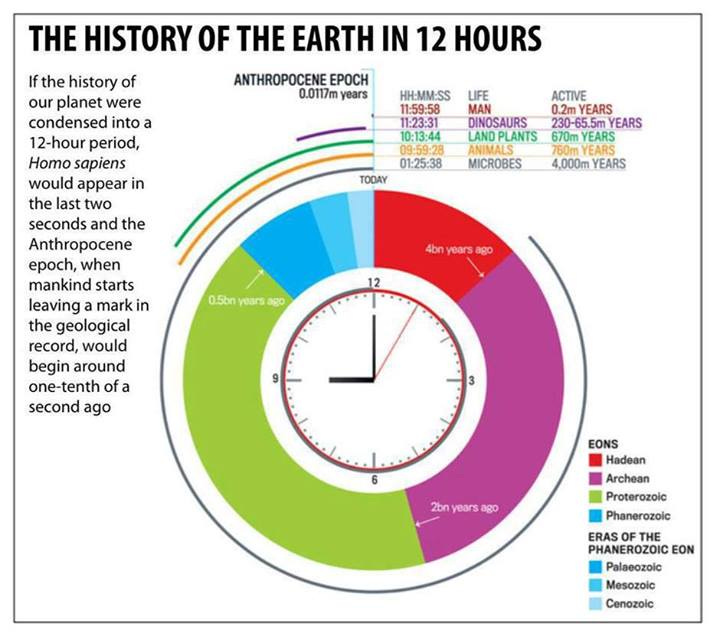
By stepping back and looking at the big picture, it’s quite easy to see the rate of effect we are having on the planet. We are warming the planet 100 times faster than at any time in history. We don’t need to argue about carbon emissions and whether or not we can impact the environment, when we stand back from the 10,000 foot perch, we don’t need to know anything about carbon footprint to see the massive impact we are having globally.
And now let’s talk about feedback loops, and how they play with changes like this. There are two types of feedback loops--negative feedback and positive feedback loops. In negative feedback loops, you see a reverse in the loop elements. For example, your room gets hot, your thermostat turns on, the A/C turns on, the room gets cold, the thermostat turns off, the A/C turns off, and so on. Negative feedback is good for stasis-- our bodies run on negative feedback, things that need to stay in a band do well with negative feedbacks. It’s self regulating. Negative feedback, however, is not great for systems trying to grow.
Positive feedback does the opposite. Positive feedback is when you’re continuing to amplify the original action-- think about a microphone loop at a concert-- no one loves that. Positive feedback loops aren’t always obvious however, because often times they’re growing, and growing, and growing, and then they finally overcome another negative feedback loop. The hollowing out of the American middle class is a perfect example of a positive feedback loop; as middle-class America was slowly hollowed out through a positive feedback loop of tax restructuring, free trade agreements, and union dissolutions, and pay flatlined, the purchasing power of the middle class slowly eroded to the point that marginal cost inflation of things like healthcare are now 10% increases on salaries, because the salaries didn’t keep up.11 Positive feedback loops quickly become exponential.
The moment the system flips from a positive feedback loop is called bifurcation. Sometimes, bifurcation is good. Sometimes, it’s not. So what does this have to do with what we’re talking about, climate change? Right now, the arctic temperature is warming, which means the ice is melting, and less ice means less reflection of sunlight, which is called the albedo effect. Now, your water temperature is going up since there’s less ice, helping melt more ice, reinforcing the arctic temperature increases. Additionally, as arctic temperature increases, permafrost melts. Methane increases, which means heat trapping increases, also further increasing arctic temperatures. The question is, when will we hit bifurcation? And what will happen from there? We may enter a new positive feedback loop, or we may have a stasis.
The Laws of Thermodynamics and Climate Change
Ok, so that makes sense, right? Let’s flip to the second law of thermodynamics. You might remember this from high school science-- no energy can be created or destroyed-- all of the energy of the universe today is the same exact amount as there was 100,000 years ago. That said, we can still transform it, whether it’s gas being turned into heat or water running downstream. Now, part of this is that none of the energy converts 100%-- there are inefficiencies-- usually exhibited by heat or light. It sounds like it’s not a big deal, but it is.
There are three forms of this, and the first one is called anti-entropic, which is when something takes in more energy than it puts out. Anything growing-- a baby, a tree, a piece of grass, is considered anti-entropic. It is storing energy, and is usually tied to positive feedback loops. As these things reach maturity and take in equal to what they take out, they’re called dynamic equilibrium. Like most adults. We aren’t getting bigger, at least not on purpose, and we are in a stasis. The last is an entropic system when systems lose more energy than they take in. Usually this is the beginning stages of decay and collapse. Entropic systems go from complexity to simplicity, and become diffusive.
So let’s talk about entropy in particular. Decay and collapse is interesting because this is where we see energy transformed, for example in the breakdown and degradation of the complex systems like the nutrient cycles in the soil, when rainforest is cleared for soy crops these systems are destroyed or the burning of concentrated forms of energy like oil into heat, energy, and fumes-- in both of these very simple examples we see entropy play out as complex resources are divided into the sum of their parts, which, if we recall, from complex systems, are less than they are as a whole.
Now all this-- this is why our current system is not sustainable. All environmental problems are forms of entropy, and when you start to think about it, it’s totally obvious.12 Whether it’s your monoculture grass, which needs intense amounts of fertilizer and weed killer to be aesthetically pleasing, or the intensive need of potash for fertilizers for our food supply, or the dwindling availability of fish within the ocean-- all of these are entropic processes, and removing diversity and energy from these systems causes more damage than just the individual piece that is removed, because, again, the value of the complex system is more than the sum of its parts.
So let’s go back to that 10,000 ft view again. Life has existed on the planet for about 3.8 billion years. For the first 3.5 billion years, the earth was an anti-entropic system.13 The planet was taking in more resources from the sun than it was releasing. This is what our soil is built of, our fossil fuel reserves stem from, the buildup of molecules in our air like oxygen, and so on. Then, about 300 million years ago, we bifurcated and entered a dynamic equilibrium. But, for the first time in the last 3.8 billion years, and I cannot emphasize this enough, we are entering into an entropic system.14 We are actually losing 10% of the energy coming to the sun and sending it back into outer space, which, at first glance might seem like a good thing.15 Isn’t getting rid of the sun’s heat good for global warming? Well the thing is, we are becoming more and more entropic, as we continue degrading the complex systems further and further as our energy use continues to rise.
With this framework, we can start to see how solutions to climate change today aren’t addressing the complex systems science that underscores climate collapse. Addressing individual issues, whether it be plastic waste, carbon in the atmosphere, or any other isolated marker. In the next section of these posts, we’ll explore this further and continue to explore the ways in which ecology, complex systems science, agriculture, economics, and climate change are all intertwined, and require an equally interconnected solution.
If you’ve enjoyed this piece, which is equal to a 10 page chapter, of (so far) a 10 page book with 15 sources, you can support our work a number of ways. The first is by sharing this article with folks you think would find it interesting. The second is by listening and sharing the audio version of this content, the Poor Proles Almanac podcast, available wherever you get your podcasts. If you’d like to financially support the project, and get exclusive access to our limited paywalled content, you can subscribe on Substack or Patreon, which will both give you access to the paywalled content, and in the case of Patreon, early access to the audio episodes as well.
Hanne Andersen, centuries, A. F., Driesch, H., Smart, J. C., & Nagel, E. (2002, January 15). The history of reductionism versus holistic approaches to scientific research. Endeavour. Retrieved February 2, 2023, from https://www.sciencedirect.com/science/article/abs/pii/S0160932700013879
https://asc-cybernetics.org/foundations/history.htm
Poincaré, J. Henri (2017). The three-body problem and the equations of dynamics : Poincaré's foundational work on dynamical systems theory. Popp, Bruce D. (Translator). Cham, Switzerland: Springer International Publishing. ISBN 9783319528984. OCLC 987302273.
Waldrop, M. M. (1993). Complexity the emerging science at the edge of Order and Chaos. Viking.
Wessels, Tom (2023). Myth of progress: Toward a sustainable future. BRANDEIS UNIV PRESS.
https://www.climate.gov/news-features/climate-qa/whats-coldest-earths-ever-been#:~:text=Striking%20during%20the%20time%20period,still%20in%20an%20ice%20age.
https://news.arizona.edu/story/global-temperatures-over-last-24000-years-show-todays-warming-unprecedented
Wessels, T. (2016). Simply Complex Systems. TedX Talk. Windham; NH.
https://climate.nasa.gov/news/2948/milankovitch-orbital-cycles-and-their-role-in-earths-climate/#:~:text=Earth's%20axis%20is%20currently%20tilted,about%209%2C800%20years%20from%20now.
https://www.climate.gov/news-features/understanding-climate/climate-change-global-temperature
https://www.ama-assn.org/about/research/trends-health-care-spending
https://www.ecologycenter.us/population-dynamics-2/entropy-and-life.html
https://history.nasa.gov/CP-2156/ch4.8.htm
Gan, Z., Yan, Y., & Qi, Y. (2004). Entropy budget of the Earth, atmosphere and ocean system. Progress in Natural Science, 14(12), 1088–1094. https://doi.org/10.1080/10020070412331344851
Wessels, Tom (2023). Myth of progress: Toward a sustainable future. BRANDEIS UNIV PRESS.
Hey there! In addition to the works cited in the endnotes, do you all have any reading recommendations on chaos theory/complex systems?
This is really good, I got a lot out of this, especially the earth cycles. Thank you